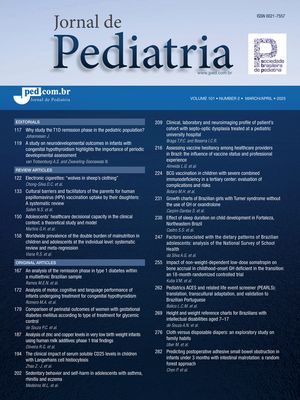
To identify and describe learnings from past pandemics and to suggest a framework for vaccine development as part of epi/pandemic readiness.
Source of dataArticles/ reviews/letters on pandemic preparedness/ vaccines published between 2005 and 2022 in PubMed, MEDLINE, MedRxiv, BioRxiv, Research Square, Gates Open Research; who.int, cepi.net, visualcapitalist.com, airfinity.com, ted.com websites; press releases.
Summary of findingsDisease pandemics caused by emerging pathogens impacted the social development, health and wealth of most societies in human history. In an outbreak, the first months determine its course. To block an exponential spread and the development of an epi/ pandemic early, vaccine availability in sufficient quantities is of paramount importance. It is inevitable that new human viruses will emerge. Any future pandemic will come likely from RNA viruses through zoonotic or vector transmission, but we cannot predict when or where “Disease X” will strike. Public health, scientific and societal readiness plans need to include: continuous identification of new viruses in common mammalian reservoir hosts; continuous epidemiological surveillance, including wastewater sampling; establishment of prototype vaccine libraries against various virus families sharing functional and structural properties; testing of various and innovative vaccine platforms including mRNA, vector, nasal or oral vaccines for suitability by virus family; functional clinical trial sites and laboratory networks in various geographies; more efficient phasing of preclinical and clinical activities; global harmonization and streamlining of regulatory requirements including pre-established protocols; and societal preparedness including combating any pandemic of misinformation.
Conclusions“Outbreaks are unavoidable, pandemics are optional”.
Disease epidemics caused by emerging pathogens have impacted most societies in human history. Both the death toll and the impact on development, health, and wealth were dramatic. The disease that most impacted humankind was the bubonic plague or “Black Death”, invoking direct comparisons to our current coronavirus “modern plague.” There were at least 3 pandemic waves caused by Yersinia pestis: the Plague of Justinian which erupted in 541 AD in Egypt: ‘‘none of the lands bordering the Mediterranean escaped it’’. The second pandemic originated most likely from Central Asia, arrived via Genoese trading ships carrying flea-infested rats in Sicily in the autumn of 1347, circumnavigated most of continental Europe in less than three years, and eventually reached places as remote as Greenland. That wave devastated Europe: based on community records it is estimated that between 1347-1352 over 25 million people – at least one-third of Europe's population - died during that pandemic. It took Europe more than 200 years to recover its population. This second Yersinia pestis pandemic lingered around in Europe for five centuries. It caused periodically massive outbreaks like the ones in London, Vienna, and as far as Moscow in the 17th /18th centuries, each causing about 100.000 fatalities. The third “Black Death” pandemic started in China and ravaged India before traveling westwards by sea. It was mostly confined to coastal cities with a modest death toll. Yersinia pestis did not only cause devastating pandemics but, as it can be easily weaponized, it is also a current threat as a bioterrorism agent.1,2
Disease epidemics and pandemics were always related to trade, migration, and the ever-increasing human invasion of the habitat of wild living animals. It is thus not surprising that the intervals between pandemics are shortening. Since the Spanish Flu in 1918, 8 epidemics/ pandemics caused by different pathogens were recorded, almost the same number as recorded since the Antonine Plague in the second century. Moreover, 5 of those 8 pandemics post Spanish Flu occurred in the last 2 decades (Table 1).3
Major epi/pandemics over time.
Source: Adapted from LePan.3
Abbreviations: COVID: coronavirus disease; HIV/AIDS Human Immunodeficiency Virus/ Acquired Immunodeficiency Syndrome; MERS: Middle East Respiratory Syndrome; SARS: severe acute respiratory syndrome.
Outbreaks, epidemics, and pandemics in the past used to be democratic. Treatments or vaccines were unavailable. However, social distancing and quarantine were adopted early as potentially effective measures for individual and societal protection. The practice of quarantine began during the 14th century to protect coastal cities from the plague imported by sailors. In Venice, ships arriving from infected geographies had to anchor 40 days, “quaranta giorni”, outside the city before disembarkation. The only other form of protection was wearing protective gear such as masks. The State of Venice required its citizens and visitors to the Republic to don masks in many public spaces till the 18th century.
An example of the democracy of viruses in the past is smallpox – it killed ordinary citizens and the extraordinary alike (Table 2).4
Sovereigns killed by smallpox.
The newest epidemics – the COVID-19 pandemic, the severe acute respiratory syndrome (SARS) pandemic, and the Swine Flu pandemic - were all undemocratic: no equal access to vaccines, diagnostics, protective gear, or treatments between countries. Within many countries, access and acceptance of measures depended on social class and ethnicity. At the height of the COVID-19 pandemic, Canada had secured 9 vaccine doses/ citizen, Brazil 1 dose, and the global facility Covax was left with less than 0.2 doses to supply the least developed countries. Even in the 3rd year of the pandemic, there are huge inequalities. In Latin America, as many as 93% of Chileans and 95% of Cubans have received at least one dose of vaccine, but as few as 2.2% in Haiti or 29.2% in Jamaica.5 Given the significant relationship between vaccination rates and GDP growth, many of the poorest countries with low vaccination rates experience in addition a more profound negative effect on their economy.6
The human toll of the COVID-19 pandemic is humiliating. Reported deaths caused by SARS-CoV-2 total 6.8 million victims worldwide.5 However, the full impact of the pandemic has been even greater than the reported COVID-19 deaths. Comparing the annual all-cause mortality in the first 2 years of the pandemic to the last 10 years pre-pandemic shows excess mortality directly or indirectly caused by SARS-CoV-2 of 18.2 million (95% CI: 17.6 – 19.6).7 The global economic toll is equally mind-boggling: an estimated 400 million people lost their jobs due to the pandemic, and the global economic loss till 2025 is estimated by the International Monetary Fund (IMF) to amount to 28 trillion US$.
The COVID-19 pandemic, as terrible as it has been, is by far not the worst imaginable scenario when it comes to outbreaks and pandemics. The SARS-CoV-2 virus is one of the most transmissible viruses ever recorded. The rate of new mutations is unexpectedly high and gives the variants an advantage through either immune evasion or further increased transmissibility. It is estimated that the ancestor strain had an R0 of 3.3, that of Omicron BA.1 was already of 9.5, but of Omicron BA.5 and BA.2.75, of 13-18, which brings these close to the transmissibility of measles. The newest Omicron subvariants XBB or BQ1.1 do not appear to be more transmissible, but seem to have an increased evasion potential. SARS-CoV-2 is not as lethal as other pathogens with pandemic potential. The coronaviruses that caused SARS and the Middle East Respiratory Syndrome (MERS) are 20 and 70 times more lethal. A nightmare scenario for mankind would be a naturally evolving or human-made virus with the transmissibility of SARS-CoV-2 and the lethality of SARS, MERS, or the Nipah virus.
The beginning of the COVID-19 pandemic is reminiscent of the times when smallpox, measles, rubella, polio or influenza were spreading unexpectedly and rapidly, some of them causing outbreaks with high mortality. These were the times when no vaccines were available. Scientific breakthroughs, novel manufacturing platforms, and exponential growth in the numbers of newly developed and deployed vaccines in the last few decades have resulted in the control of many infectious diseases including some with pandemic potential.
Unfortunately, targeted research on diagnostics, treatment, and vaccines for pathogens with pandemic potential was widely neglected by industry because of lack of a commercial opportunity and by academia because of a lack of funding. The tipping point in the global approach to pandemic threats was the 7th Ebola outbreak in Western Africa in 2014/2016. This outbreak was 10 times larger than all the previous 6 outbreaks combined - more than 28.600 people were infected and over 11.300 died. The societal financial burden was of over US$53 billion, of which one-third arrived from the impact on the health care system. About 10% of the healthcare workers died, causing an increase in deaths from non-Ebola diseases such as cancer and other non-communicable and infectious diseases.8
The world's response to this crisis fell tragically short. An Ebola vaccine that had been under development for more than a decade was not licensed mainly because of regulatory hurdles, and this emergency vaccine was not deployed until over a year into the epidemic. The vaccine was shown later to be virtually 100% effective against the Zaire strain, suggesting that much of the epidemic could have been prevented.9
Following global criticism about the inadequate and delayed response of society to the 7th Ebola outbreak, various initiatives were launched for reforms in response to the next pandemic. These were mainly driven by scientists and philanthropy, and not by public health stakeholders.10,11 Averting a repetition of this failure was the motivating factor behind founding CEPI, the “Coalition for Epidemic Preparedness and Innovation” which is based in Oslo, London, and Washington DC. CEPI is a global partnership between the public and private sectors, philanthropy and civil society. It was formally launched at the World Economic Forum (WEF) in Davos in January 2017 – not at the World Health Assembly or other Global Health stakeholder meetings which are telling. Initial founders and funders were the governments of Norway, Japan, Germany, and the UK, as well as the Bill & Melinda Gates Foundation and the Wellcome Trust. With an initial funding target of 1 billion US$ for 5 years, CEPI is by far the largest vaccine development initiative ever against viruses that can potentially cause epidemics/ pandemics.12
CEPI's mission is to accelerate the development of vaccines and other biologic countermeasures against epidemic/ pandemic threats whilst ensuring equitable access globally. The initial stretch goal was to shorten the development time of a vaccine from more than 10 years to 1-2 years. CEPI operates according to 2 concepts:
- -
Advancing vaccines against known pandemic threats through preclinical development, proof-of-concept, and safety testing in humans till phase II, and by establishing vaccine stockpiles: ”Just In Case”.
- -
Funding platform technologies including mRNA and viral vectors to accelerate the development and manufacturing of vaccines against previously unknown pathogens, the “Disease X”: ”Just In Time”.
- -
Known emerging, re-emerging or unknown infectious pathogens “spilling over” from an animal reservoir to humans – similar to SARS-CoV-2, MERS, and other coronaviruses. Evidence is increasing that SARS-CoV-2 indeed jumped from animals sold at the Wuhan market in China on probably two occasions in late 2019.13,14
- -
Climate change and deforestation: ecological changes pose an enormous and underappreciated threat to the occurrence of outbreaks and pandemics. The natural habitat of wildlife is continuously diminished and urbanization is accelerated, bringing wild animals such as bats or monkeys serving as a potential virus reservoir closer to humans and thus increasing transmission risk – Ebola, Nipah, or urban Yellow Fever. Climate change itself is seen as the most important reason for the expansion of vector-borne diseases such as Dengue and Chikungunya to further North and South. The mechanistic connections between climate change, habitat loss, and viral spillover risk were recently demonstrated in a long-term Australian ecological study of flying foxes. These bat-like animals are host to the Hendra virus – a paramyxovirus like the Nipah virus – which can cause in humans severe respiratory infections with up to 50% lethality. The Hendra virus was identified in 1994 when it spread from its flying-fox reservoir to horses through excrements and spats causing outbreaks in horses which then served as an intermediate for human infections. Flying foxes shed more virus and consequently trigger more Hendra spillover infections in years in which they experience food stress. These food shortages typically follow years with a strong El Niño, the climatic phenomenon in the tropical Pacific Ocean that can cause droughts affecting, in Australia, the native eucalyptus tree budding which is sensitive to climate changes. Flying foxes normally live a “nomadic” lifestyle - moving in large groups from one native forest to another in search of food. However, when food is scarce like in the post-El Niño winters, they split into small groups and settle in urban and agricultural areas in the proximity of horses and people. To conserve energy, the bats fly only small distances, scavenging for food in agricultural areas near horses and humans and thus increasing the spillover risk. However, when the trees in the native forests the bats rely on for food have a large flowering in the winter after a food shortage, mass migrations take the flying foxes back to these forests, away from horses and humans, and the risk of spillovers is largely reduced. Thus, restoring the natural habitat for hosts of potentially lethal viruses reduces the risk of spillovers and protects humans and animals alike.15
- -
“Spilling back” of pathogens from infected humans to animals which then serve as reservoirs for new animal-human transmission – “reverse zoonotic transmission”.16
- -
Lack of adequate public health infrastructure for early detection of outbreaks, pathogen sequencing, early containment, community education and engagement, vaccination and treatment campaigns, and combating fake news epidemics.
- -
Acts of bioterrorism.
- -
Development of antimicrobial resistance.
Most classes of microbes including bacteria could evolve or be manipulated with a catastrophic risk to humans. Whilst bacterial pandemics such as the plague historically had a profound impact on human society, the risk of bacteria causing pandemics is nowadays comparatively remote. The slower speed of replication and accumulation of mutations disadvantage bacteria over viruses and for most of the bacteria with outbreak potentials, such as cholera or typhoid, effective antibiotic treatments and vaccines are available. There is however an emerging public health crisis of multiple-drug-resistant bacteria such as carbapenem-resistant Enterobacteriaceae (CRE) and others. More than 1.27 million deaths/per year are directly attributable to antimicrobial resistance (AMR).17 Industry has scaled down research in novel classes of antibiotics because of lack of profitability to the extent that, since 1990, not a single novel antibiotic class was developed or patented. The “Combating Antibiotic-Resistant Bacteria Biopharmaceutical Accelerator” (CARB-X) is a global non-profit partnership established and supported by the Wellcome Trust, BARDA and various western governments to fill this gap and to accelerate the development of antibacterial compounds to address drug-resistant bacteria. The CARB-X portfolio is the world's most diverse early development pipeline of new antibiotics, novel and repurposed vaccines, rapid diagnostics, and other products.18
There is consensus that the next outbreak which could turn into a pandemic will most likely be caused by a virus. RNA viruses represent a higher pandemic threat than DNA viruses as the stability of RNA is less than that of DNA, thus facilitating mutations.19,20 The virus universe is immense: more than 100 virus families have been described and around 260 viruses from 25 families are known to infect humans (Figure 1). There are licensed vaccines for viruses within 13 of these families. Another 1.6 million viruses from the 25 families are thought to exist in mammals or bird hosts/ reservoirs of which up to 50% are thought to be able to infect humans.21-24
For a pathogen to have the capacity to cause a pandemic it needs several attributes: effective mode of transmission, human-to-human transmission, and lack of specific countermeasures as the most important. Of the various transmission modes, the respiratory route, especially via aerosol, is the most efficient mechanism facilitating a pandemic's spread.19 As an example, the Alpha, Delta and Omicron SARS-CoV-2 variants of concern which are associated with increased transmissibility have a significantly higher viral aerosol shedding than the Beta or Gamma variants which did not increase in transmission.25
There are several viral families that possess RNA genomes and are spread by the respiratory routes – candidates with pandemic potential:
- -
Orthomyxoviruses: these include the influenza viruses that caused various pandemics in the last decades, among them the H7N9 influenza A strain. The recent swine Flu pandemic demonstrated the dilemma of lack of preparedness: although the manufacture of seasonal Flu vaccines is a standard process in the industry, no company was prepared for immediate pandemic Flu vaccine production, and there was even competition between the production of the pandemic or seasonal Flu strains. Thus, more than 40% of the deaths in the first wave had already occurred before meaningful amounts of pandemic Flu vaccines were available despite there being huge Flu vaccine manufacturing capacities in place. There was also a huge inequality in access.
- -
Paramyxoviruses: including the highly contagious measles and mumps viruses; and the zoonotic Henipaviruses Hendra and Nipah with high lethality.
- -
Coronaviruses: the common cold coronaviruses are widespread, easily transmissible, and contagious but typically cause only mild disease. However, coronaviruses are well known for decades to have pandemic potential. They already jumped three times from animals to humans causing pandemics: SARS and MERS which both had a high case-fatality rate but could be controlled, and the SARS-CoV-2 causing COVID-19. Despite the knowledge about the pandemic potential, there was no commercial interest in coronavirus vaccine/ treatment development and little academic research: in 2019, a mere 1,000 academic articles were published on coronaviruses – three years later, this had increased to 250,000.26
- -
Arenaviruses such as the Lassa virus are spread by rodents. Humans are mainly infected through mucosal exposure to aerosols of rodents’ dried excretion such as urine.
The second category of viruses with pandemic potential, despite mostly lacking direct human-to-human transmissibility, is vector-borne viruses. The geographical footprint of the mosquitos serving as vectors for these viruses is ever-increasing due to climate changes and hence the risk that these viruses move from a regional outbreak to an epidemic or even pandemic situation is also increasing.
- -
Flaviviruses such as Yellow Fever, Dengue, and Zika. Vaccines are available for Yellow Fever though often out-of-stock situations occur due to a very small number of suppliers. Two Dengue vaccines were licensed but with limited indications. For Zika, the most advanced vaccines are in phase II.
- -
Togaviruses such as Chikungunya, Venezuelan encephalitis, and the Mayaro virus. Facilitated by CEPI funding, impressive progress has been made in the development of Chikungunya vaccines.
Lastly, Filovirus family members can cause highly lethal outbreaks and epidemics. The first filovirus, detected 45 years ago, was the Marburg virus after several laboratory workers in Marburg, Frankfurt and Belgrade came up with unexplainable severe hemorrhagic fever.27 The Marburg virus is highly lethal and causes sporadic outbreaks like the one in June 2022 in Ghana, but, so far, no epidemics.28 The Filovirus family includes also the Ebola virus with its Zaire, Sudan and Bundibugyo strains. Whilst there is no specific vaccine for the Marburg virus, two vaccine schemes against Zaire Ebola showed very high efficacy: a recombinant vesicular stomatitis virus vector – the Zaire Ebola virus vaccine (rVSV-ZEBOV), and a scheme consisting of the first dose of an adenovirus-vectored Ad26.ZEBOV vaccine followed by a second dose 8 weeks later of an MVA-vectored vaccine - MVA-BN-Fil. This vaccine is not protective against the Ebola Sudan strain subtype causing the current outbreak in Uganda. Despite having the experience and blueprint to make and license Ebola vaccines, industry efforts to develop proactively a Sudan or Bundibugyo-adapted vaccine stalled for lack of incentives and abundant bureaucracy. Thus unfortunately industry and global society are once more failing in the combat of Ebola epidemics despite the tools available.
The most important emerging and re-emerging pathogenic viral infections in the last 20 years are depicted in Figure 2. Whilst it is impossible to develop vaccines against all potential viral threats, a promising strategy could be to establish a library of prototype vaccines, monoclonal antibodies, and repurposed licensed pharmaceuticals against representative viruses from the 25 viral families implicated in human disease.
Virus families and species have commonly shared functional and structural properties. Thus it can be postulated that vaccine approaches established for a prototype within a family would be functional also for other closely related family members. As part of this concept, scientists would establish appropriate animal models, and immunological readouts, including validation of assays, assessment of protective pathways, preclinical studies including challenge trials, clinical studies up to phase I/II and a manufacturing blueprint. Ultimately, it might be possible to even establish the appropriateness of various vaccine platforms for each viral family or species.
One issue in the development of vaccines against diseases with epi/pandemic potential is that it is difficult to impossible to perform pre-licensure field efficacy trials because the occurrence of outbreaks is unpredictable. Therefore a regulatory debate is ongoing to license such vaccines based on correlates by comparing vaccine-induced antibody/ CMI levels to those in people protected after natural infection, or through the animal route wherein validated experimental model animals are vaccinated and challenged. Regulatory agencies could grant EUA/ licensure with a condition of approval that the applicant has clinical efficacy/ effectiveness protocols ready for an immediate study start as outbreaks occur.
CEPI initially selected and supported vaccine development against 5 pathogens identified as a potential risk for a pandemic based mainly on the WHO R&D Blueprint list – the “just in case” approach.29 CEPI considered in addition as an additional criterion market failure due to lack of commercial interest by the industry. The initial CEPI funding included vaccines against 5 viruses with a total of 21 different vaccine candidates against:30
- -
Nipah: 4 candidates - an HeV-sG-V (Hendra Virus Soluble Glycoprotein Vaccine) and a vector-based candidate which are in phase 1, and two other vectored vaccines at the preclinical stage. A successful development of Nipah vaccines could be of particular importance given the high lethality of Nipah and the recent detection of the novel Henipavirus Langya Virus (LayV) in China.31
- -
MERS: 5 candidates - 3 viral vectors, 1 recombinant protein, and 1 DNA vaccine. The Inovio DNA candidate and the Oxford ChadOx vector candidate are at the clinical development stage.32,33
- -
Lassa fever: 6 candidates based on mRNA, DNA, and viral vector platforms. Three of those are at the preclinical stage and three are in phase 1 clinical trials.
- -
Rift Valley fever: 2 candidates, both live attenuated viral approaches at the preclinical stage.
- -
Chikungunya: 3 candidates - an inactivated whole virion candidate, measles vectored vaccine, and the live attenuated single dose vaccine VLA-1553 developed by Austrian researchers. This vaccine which is safe and highly immunogenic has been submitted for licensure based on a correlate of protection34,35 and not on a prelicensure efficacy trial. The vaccine has received Breakthrough Therapy and Fast Track from the FDA and PRIME designation from the EMA and should be available soon. The originator also entered into a production partnership with a Brazilian manufacturer.
CEPI leadership was of high importance in the development of vaccines against SARS-CoV-2. The critical impact of CEPI stemmed from the selection of MERS as one of the pathogens for early vaccine development funding. This helped grantees such as the University of Oxford and Inovio to jump-start the development of their chimpanzee-adenovirus vectored or their DNA-based COVID-19 vaccines, validating the prototype family vaccine concept. The ChadOx1- SARS-CoV-2 from the University of Oxford was one of the first vaccines to receive emergency use authorization worldwide within less than a year of sequence availability. This would not have been possible without the preceding MERS work. In total, CEPI supported the development of 14 COVID-19 vaccines on different platforms.
By now, more than 12.9 billion doses of COVID-19 vaccines5 have been distributed. A study by the Imperial College London, UK, estimated that in the first year of vaccination rollout about 20 million lives were saved representing a global reduction of 63% in total deaths (Figure 3).36 Based on this and through their own research, Airfinity calculated that the vaccine from the University of Oxford/ AstraZeneca/ Fiocruz accounted for over 6.3 million of the deaths averted.37 Unfortunately 96 countries and administrative regions were below the end 2021 WHO target of 40% vaccination coverage. Had this target been met, an additional 600.000 deaths could have been averted.36
COVID-19 deaths averted by vaccine.37 Available at https://www.airfinity.com/insights/astrazeneca-and-pfizer-biontech-saved-over-12-million-lives-in-the-firstl.
The SARS-CoV-2 virus will not disappear but will mutate again. The speed of these mutations and their transmissibility/ evasion characteristics versus the speed to develop novel vaccines protecting across current/ future unknown variants and a prolonged duration of protection will determine the endgame of this pandemic. CEPI funds 7 broadly protective COVID-19 vaccines based on various platform technologies, including novelties such as mosaic-nanoparticles, and the multi-antigen-presenting system MAPS. Other approaches are nasal/ oral vaccines to ensure mucosal immunity at the viral port of entry, as well as heterologous prime/ boost regimens with different platforms.
There are 4 endgame scenarios for COVID-19: eradication, elimination, cohabitation, or conflagration.38 Keeping in mind that only 3 viruses have been eradicated so far (smallpox, wild-type polio 2 and 3) option 1 and also option 2 are unlikely to occur. In a cohabitation situation, vaccine-mediated protection would prevent severe COVID-19, interrupt viral transmission and protect against most emerging variants. The conflagration scenario would be a steady state moderate-level endemicity of SARS-CoV-2 if important segments of the population are not vaccinated or are under-vaccinated. This would allow the virus to continuously adapt and evade host-mediated and vaccine-derived immune responses. Which scenario will prevail depends less on science and more on societal behavior.
It seems inevitable that new human viruses will continue to emerge. Science needs to be prepared for “Disease X” - an epidemic/ pandemic caused by a currently unknown pathogen. We cannot predict what “Disease X” will be, when or where it will emerge, nor the profile of such a pathogen. We even don't know what we don't know. However, the two most likely pathways from which new viruses emerge are zoonotic and vector-borne. Targeted efforts to identify new viruses in common mammalian reservoir hosts, such as bats and rodents, and in intermediate hosts, such as domesticated animals and primates, could identify future potential threats. Likewise, systematic screening of common vectors such as mosquitoes for new viruses should be done. CEPI invested in various innovative vaccine platform technologies that enable rapid vaccine development and scalability to respond to outbreaks of Disease X – the “just in time” approach. This includes different mRNA technologies, a molecular clamp platform, and a novel manufacturing approach, “vaccine printing”. This a fully automated process for mRNA manufacturing the size of a few airline containers requiring only a very small facility footprint as mRNA production is a chemical and not a biological process. Deployed to regions with outbreak potential, this approach could ensure very rapid local availability of clinical trial materials to allow for quick study start and down selection of vaccine candidates. As an aside and the other end of the spectrum – this concept is also ideal for individualized oncological therapies. There are currently two German companies – BioNTech and Curevac jointly with Tesla – working on this concept and prototypes could be licensed shortly. The first deployment of BioNTechs “BioNTainer” to Rwanda is ongoing.
To be ready for the next pandemic, we need vaccines even quicker. Whilst the typical vaccine development timelines of 10 years were reduced to just 307 days in the COVID-19 pandemic, 70 million COVID-19 cases and 1.6 million deaths occurred worldwide between outbreak onset and first vaccinations.39 The first 100 days of an outbreak are crucial to changing its course and preventing it from becoming a pandemic. Therefore, the UK government launched, at the last G7 meeting, a “moonshot mission” – compressing vaccine development and ensuring production at scale within 100 days of the genome sequence. This is possible only if there are vaccine prototype libraries and optimized rapid response platforms. It is further imperative that clinical trial sites and laboratory networks in multiple geographies are available and functional with adequate infrastructure and trained staff to ensure rapid start and study conduct in quality.40 To ensure readiness these sites need a continuous flow of other vaccine trials outside a pandemic to maintain their capabilities. Substantial time savings need to come from the more efficient phasing of preclinical activities, seamless clinical trials, global harmonization, and streamlining of regulatory requirements including pre-established protocols.
Whilst early vaccine availability is a core element of containment when an epidemic strikes, the key to avoiding future epidemics and pandemics is early detection of local or regional outbreaks and identification of the causative pathogen. To avoid sleepwalking into the next pandemic, it is imperative that countries maintain disease surveillance capabilities after COVID-19. An important tool is wastewater surveillance which detects pathogens early, in the case of SARS-CoV-2 VoCs, 2 weeks before the first positive nasal swabs in a community.41 Accurate and approved rapid point-of-care diagnostic tests need to be available in the same 100-day time horizon as vaccines as part of the moonshot ambition, as well as disease and transmission modeling capabilities for prediction of the course of a pandemic. Society needs to be much better educated and prepared than in the current COVID-19 pandemic, and there need to be global and local strategies on how to address the misinformation and fake news pandemic. A recent coherent proposal for pandemic readiness suggests an “outbreak fire brigade”: a full-time Global Epidemic Response and Mobilization (GERM) team of scientists of various backgrounds based in regional WHO offices to detect, monitor and alert.42
Early detection is key: “Outbreaks are unavoidable, pandemics are optional”.4
The authors want to thank Celine Pompeia and Regina Sider (Intrials, Sao Paulo, Brazil) for their help in preparing the manuscript.