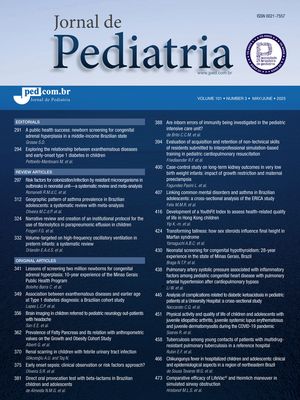
Since the beginning of its use for the prevention of tuberculosis (TB) in 1921, other uses of BCG (Bacillus Calmette-Guérin) have been proposed, particularly in the treatment of malignant solid tumors, multiple sclerosis, and other autoimmune diseases. Its beneficial impact on other infections, by nontuberculous mycobacteria, and by viruses, has been more often studied in recent years, especially after the introduction of the concept of trained immunity. The present study's objective was to review the possible indications of BCG and the immunological rationale for these indications.
Data sourceNon-systematic review carried out in the PubMed, SciELO and Google Scholar databases, using the following search terms: “BCG” and “history”, “efficacy”, “use”, “cancer”, “trained immunity”, “other infections”, “autoimmune diseases”.
Data synthesisThere is epidemiological evidence that BCG can reduce overall child morbidity/mortality beyond what would be expected from TB control. BCG is able to promote cross-immunity with nontuberculous mycobacteria and other bacteria. BCG promotes in vitro changes that increase innate immune response to other infections, mainly viral ones, through mechanisms known as trained immunity. Effects on cancer, except bladder cancer, and on autoimmune and allergic diseases are debatable.
ConclusionsDespite evidence obtained from in vitro studies, and some epidemiological and clinical evidence, more robust evidence of in vivo efficacy is still needed to justify the use of BCG in clinical practice, in addition to what is recommended by the National Immunization Program for TB prevention and bladder cancer treatment.
Since the beginning of its use in the prevention of tuberculosis (TB) in 1921, other uses of BCG (Bacillus Calmette-Guérin) have been proposed in the treatment of solid malignant tumors and, more recently, in the treatment of multiple sclerosis and other autoimmune diseases. Its beneficial impact on other infections, both by nontuberculous mycobacteria and by viruses, has been often more studied in recent years, especially after the introduction of the trained immunity concept.1 This concept represents a paradigm shift in immunology and is related to the ability of innate immunity to develop memory, although less specific and long-lasting than adaptive immunity.2
The objective of the present study was to review the possible indications of this century-old vaccine and the immunological rationale for these indications. A non-systematic review was carried out on the use of the BCG vaccine, here in presented in three parts: a) data on the vaccine itself, its efficacy and immune response; b) trained immunity, concept, and BCG action; c) BCG uses beyond TB prevention. The Pubmed, SciELO, and Google Scholar databases were used for the search, with the following search terms: "BCG" and "history", "efficacy", "use", "cancer", "trained immunity", "other infections", " autoimmune diseases”.
The BCG vaccineThe BCG vaccine consists of a live attenuated strain of Mycobacterium bovis and was used for the first time in 1921. It is the only currently licensed vaccine for human use to prevent TB,3 with variations in the used strains and vaccination practices among the countries that use it.4
To prevent the accumulation of genetic changes in the strains, the World Health Organization (WHO) recommended, in 1966, the freeze-drying of the seed lots used in the preparation of vaccines. In this scenario, the production of each lot starts with the same lyophile, the product being monoclonal.5 The main strains currently used for vaccine production are the Brazilian (Moreau/Rio de Janeiro), the Danish (Glaxo and Copenhagen – 1331), the Japanese (Tokyo – 172-1), the Russian (Moscow – 368), and the Bulgarian (Sofia - SL222) strains.6
In Brazil, BCG vaccination was implemented in 1925, using the BCG-Moreau strain, administered orally until 1973 and, thereafter, intradermally.5 The BCG Moreau-RJ vaccine, manufactured by Fundação Ataulpho de Paiva (FAP) was used in Brazil until February 2018, when, due to its manufacture interruption, the National Immunization Program (PNI) had to purchase a vaccine from another laboratory. The purchased BCG vaccine is manufactured by the Serum Institute of India, according to information on the package insert, using the Russian BCG strain (Moscow-368), of the same strain as the Moreau-RJ vaccine.7
BCG efficacyThe protective efficacy of the BCG vaccine against TB varies substantially between studies and is associated with protection against disseminated TB in childhood (meningitis and miliary TB), but does not satisfactorily prevent pulmonary TB or its transmission.8 There is a range of factors that can interfere with its efficacy.9 The absence of a previous M. tuberculosis infection or sensitization by nontuberculous mycobacteria (NTM), also called environmental mycobacteria, is strongly associated with greater BCG efficacy against pulmonary tuberculosis and possibly against miliary and meningeal tuberculosis. On the other hand, in tropical regions, the more prevalent helminth infestation has been suggested as being responsible for limiting vaccine efficacy.9
Disseminated tuberculosis disease has its peak in the first year of life, which is why vaccination at birth (or at first contact with health services) is recommended in countries where tuberculosis is endemic.3 There is little evidence that revaccination with BCG offers increased efficacy or duration of protection.6 However, individuals revaccinated in adulthood have demonstrated that BCG revaccination significantly increased Th1 and Th17-type responses in those with or without prior contact with Mycobacterium tuberculosis.10
It is estimated that BCG can prevent more than 17,000 deaths from tuberculosis in the first fifteen years of life.11 There is also a growing number of authors in the literature who propose non-specific benefits of BCG vaccination, including a reduction in infant mortality from all causes and protection against unrelated infectious diseases,12 a topic to be addressed later in this text.
Immune response to BCGThe different sectors of the immune system act in response to infectious agents; however, the involved cells and soluble components vary primarily according to the type of microorganism. Mycobacteria are intracellular pathogens and, in this case, the immune response occurs primarily by phagocytosis with intracellular destruction (innate immunity) potentiated by Th1 lymphocytes (adaptive immunity).13 In the case of a vaccine consisting of a live mycobacterium, the response to BCG occurs in the same way.
BCG vaccination triggers the expression of different cytokines similar to those induced by infection with Mycobacterium tuberculosis or wild-type M. bovis, bacteria that have 99.95% similarity in their genomic sequences.14 However, the immune response to the BCG vaccine has some peculiarities, such as the different routes of entry (intradermal versus inhaled)14 and the genetic changes that occur with attenuation. For instance, BCG does not have a locus called ESX-1 which, although it offers sufficient attenuation compared to M. bovis or M. tuberculosis to allow its use as a vaccine, prevents phagosome disruption, cytosolic contact and host cell death after uptake by antigen-presenting cells. Hence, there are several cytosolic detection systems that are underutilized by the vaccine and may represent routes for inducing immune responses with greater protection.15 These differences show a protective effect that is inducible by the BCG vaccine, as opposed to the immune response to wild-type mycobacteria.8,16
The initial immune response to BCG occurs at the site where it is applied. After intradermal inoculation of the vaccine, neutrophils interact with the bacillus and increase the expression of adhesion markers CD11b and CD18, FcγRs and III, and the upregulation of cytokines (interleukin [IL]-1α, IL-1β, transforming growth factor [TGF]-β, IL-8, chemokine ligand [CCL]2 and CCL3), which recruit monocytes and dendritic cells (DC) to the site. Epidermal resident macrophages and epidermal DC interact with the bacillus through different pattern recognition receptors (PRRs) expressed on its surface, mainly toll-like receptors (TLR) such as TLR2, TLR4 and complement receptor 3 (CR3), which phagocytizes mycobacteria and initiates the innate response. BCG restricted to the phagosome of the host cells induces the processing of its antigens together with the major histocompatibility complex (MHC) class II molecules.17,18
Mycobacterial-infected migratory epidermal DC migrate from the inoculation site to the draining lymph nodes. As the DC migrate, they undergo maturation, with the upregulation of costimulatory molecules (CD40, CD54, CD80, and CD86). In the lymph nodes, the DC releases cytokines such as tumor necrosis factor-alpha (TNF-α), IL-6 and ISG15 protein, but mainly IL-12p40. These cytokines will stimulate TCD4+, TCD8+, follicular T cells (Tfh), regulatory T cells (Treg), and B lymphocytes to exercise their respective functions.16 The IL12-IFN-γ (interferon gamma) axis plays a key role in the immune response to intracellular pathogens, such as mycobacteria.16,18
T CD4+ cells bind to MHC class II and differentiate into Th1, Th2 or Th17 effector cells, depending on the stimuli present in their microenvironment. After the application of BCG, T CD4+ cells differentiate mainly into Th1 cells, stimulated by IL-12p40 with a production of IFN-γ. Other T CD4+ cells, in small numbers, differentiate into Th17 cells, stimulated by IL-6 and TGF-β, and into Th2 cells, which produce IL-4.8 The T CD4+ cells differentiated into Th17 effectors seem to contribute to the granulomatous response in TB, being involved in chronic infections16 and related to immune protection against more virulent clinical isolates of M. tuberculosis.8 Among the phenotypic patterns of T CD4+ cells, central memory T cells are more likely to produce IL-2 and the effector T cells to produce IFN-γ.8
T CD8+ cells recognize peptides bound to MHC class I of DC or are directly activated by T CD4+ cells through the expression of CD40 or IL-2, becoming mature cytotoxic T lymphocytes (CTLs), capable of eliminating infected cells via granule exocytosis and programmed cell death. Together with differentiated T CD4+ cells, CTLs migrate to the site of inoculation and provide the necessary stimulation for the activation of local innate cells (macrophages and dendritic cells).8
The NK cells, when stimulated with BCG, produce IL-22, perforin, and granzyme A, inducing the lysis of monocytes infected by M. tuberculosis and improving the effector functions of T CD8+ cells, thus playing an important role in protecting against TB.8
The humoral immune response in the context of TB has been scarcely studied, which may underestimate its importance.8 Recent evidence suggests that B lymphocytes and antibodies may play a more significant role than what was previously thought.19 It is known that humoral responses are marked during active tuberculosis and it has been postulated that they could be involved in immunopathology. However, research indicates that specific antibodies can limit the spread of M. tuberculosis and even play a role in preventing infection. Some studies suggest that antibodies provide protection against intracellular pathogens by targeting innate immune antimicrobial activity via opsonization and “Fc receptor”-mediated phagocytosis. These receptors are found on all innate cells and indicate a role for antibodies in the effector functions of infection control, demonstrating that humoral immunity can modulate the pathogenesis of TB.19 Thus, it is understood that the humoral response to BCG vaccination is heterogeneous and may play different roles depending on the individual and population.8,19
New studies suggest that protection against intracellular pathogens is not solely dependent on the cell adaptive response, as the response to extracellular microorganisms is not exclusively humoral. The need for both cell-mediated and antibody-mediated immunity to act in the development of an effective response to pathogens, both intracellular and extracellular, is increasingly recognized, with sophisticated cooperation between innate and adaptive responses. B lymphocytes can modulate the T cell response via cytokine production and participate in T cell priming by capturing and presenting antigens.20
Nonetheless, unlike most currently available vaccines, whose protective efficacy is assessed by the presence of specific antibodies, in the case of BCG, protective immunity basically depends on the induction of cell immunity,21 although its protection has not yet been attributed to a defined population of memory cells. It is difficult to differentiate, particularly in endemic regions, whether the long-term memory cells found are due to a history of BCG vaccination or frequent exposure to environmental mycobacteria.8
A recent study carried out in South Africa recruited more than 5,000 children vaccinated with BCG and followed them for two years, which allowed a more direct investigation of the disease risk correlates. In this cohort, the proportion of polyfunctional T cells in BCG-stimulated cultures was not associated with a reduced risk of developing active TB.22
The production of IFN-γ in response to M. tuberculosis antigen stimulation has been considered the gold standard of antimycobacterial immunity, but in the case of a vaccine, it refers to immunogenicity and not necessarily to protective efficacy. The lack of immunological markers that serve as correlates of protective immunity makes it difficult to predict whether the vaccine-induced immune response will result in effective protection.23
Trained immunity - concept and BCG actionThe immune system consists of a wide variety of cells and soluble components that cause an immediate response, with low specificity, to external agents, which is innate immunity, and a later response, very specific and capable of generating long-term memory, the adaptive immunity. In the second decade of the 21st century, a new paradigm emerged about the capacity of innate immunity to develop memory. This phenomenon was named “trained immunity” in 2011 by Netea et al. and consists of the capacity to generate an increased response of the innate immune system to a second infection caused by the same microorganism or by a different agent (cross- or heterologous protection).2
Initial evidence of this capacity of innate immunity to generate memory was obtained from studies with plants and invertebrates, which do not have the adaptive response structure (T and B lymphocytes, and antibodies). Some earlier studies have demonstrated the ability to produce cross-protection regardless of the participation of T and B cells in mammals, such as studies in which the application of a Candida albicans strain in rats promoted protection against another strain of C. albicans, as well as against Staphylococcus aureus, even in mice without the thymus.24,25 Although very important for the innate immune response, neutrophils are cells with rapid turnover and, therefore, are unlikely to be more directly responsible for the development of trained immunity. More recently, many studies have confirmed the participation of NK (natural killer) cells and macrophages in the development of this memory, through the action of several cytokines, particularly IFN- γ and CXCR6 chemokine.2
The exact mechanisms involved in the memory of innate immunity are not known, but there is current evidence that innate immunity is modulated by the history of individual exposure to infections, immunizations, and microbial metabolites, which can either stimulate or impair the immune response.26 Stimuli to the innate response, such as infectious agents or vaccines, promote epigenetic alterations, in a type of cell reprogramming by acetylation or methylation of histones in specific genes, modifying the transcriptional profile of NK cells and macrophages, with changes in their functional phenotypes and in the expression of cell surface molecules, activating PRRs and cytokine receptors.18,26,27 There are metabolic alterations, shifting from oxidative phosphorylation to aerobic glycolysis.1,27 These epigenetic and metabolic changes lead to an enhanced response when a subsequent infection occurs, by increasing the production of cytokines such as IL-1β, IL-6, and TNF-α28 and reactive oxygen species,20 promoting a faster inflammatory response and improving antigen recognition.18,27
A study with mice with severe T-B-NK+ combined immunodeficiency showed that those receiving BCG were protected against C. albicans, suggesting a relevant role of NK cells in the protection provided by this vaccine.18,29
Circulating monocytes with increased capacity to produce inflammatory cytokines and capacity to protect against S. aureus and C. albicans were identified in adults vaccinated with BCG. NK cells with an increased capacity to secrete inflammatory cytokines, such as IL-1 β and IL-6 after stimulation with M. tuberculosis, C. albicans, and S. aureus were also detected in adults three months after the BCG application.18,29 These data were obtained in adult human cells, but a more recent study confirmed the increase in the production of inflammatory cytokines also in infants, one month after the application of BCG.29
Although it is unclear whether the innate response memory plays any role in long-term protection,20 there is evidence of histone modification in the promoters of the TNF α, IL-6, and TLR4 genes in peripheral blood monocytes that lasted at least three months after BCG. These epigenetic alterations are mediated by NOD2 receptor signaling and resulted in the upregulation of key pro-inflammatory genes.18,28 Apparently, this cell reprogramming may involve bone marrow progenitor cells, stimulating myelopoiesis and generating trained immune cells with a greater ability to respond to infectious agents.18,27
In addition to this epigenetic reprogramming, the involvement of different cell metabolic pathways was identified, such as glycolysis, glutaminolysis, and cholesterol synthesis, which may interfere with epigenetic modifications. Therefore, epigenetic regulation is related to and coordinated by cell metabolic activity.18,27
BCG-promoted monocyte reprogramming was experimentally shown to protect against the yellow fever vaccine virus.18
The serological response to the triple bacterial vaccine, triple viral vaccine (MMR), 13-valent pneumococcal conjugate vaccine, meningococcal B vaccine, and vaccines against poliomyelitis and Haemophilus influenzae was compared in a group of children who received BCG in the neonatal period and a group who did not. Although no statistical significance was found, the response to diphtheria, tetanus, measles, mumps and pneumococcal serotypes was greater in the group of children who received BCG.30
A systematic review carried out aimed at evaluating the non-specific immunological effects produced by BCG, measles and triple bacterial (DTP) vaccines on all causes of mortality showed that the BCG and measles vaccines, but not DTP, were able to reduce the overall mortality more than would be expected by simply protecting against the infections they specifically target.31 On the other hand, another systematic review with the same objective published in the same year concluded that the heterogeneity and insufficient quality of the studies did not allow for obtaining reliable evidence that there are, in fact, clinically relevant heterologous beneficial effects produced by these vaccines.32
Uses of the BCG vaccine in addition to TB preventionThe role of BCG in the treatment of bladder cancer without muscle tissue invasion is well established and this is also a traditional indication for this vaccine.1,33 The genetic alterations of tumor cells, tumor biology, T cell activity, neutrophils, macrophages, NK cells, and dendritic cells have been studied separately and have been shown to be associated with the response to BCG therapy and cancer outcomes; however, there is no model yet to predict the response to intravesical BCG.34 Other BCG indications that have been proposed and studied over the years are related to the treatment and prevention of other infections, particularly viral ones, such as COVID-19; autoimmune diseases such as multiple sclerosis and type I diabetes mellitus, and allergic diseases such as asthma; and other types of solid malignant tumors, such as melanoma, prostate and lung cancer.1
BCG protection against other infectious agents is usually explained by T-lymphocyte cross-reactivity (heterologous immunity), as well as by trained immunity.29
Protection against nontuberculous mycobacteria, including M. leprae, is due to the presence of common antigens, although the use of BCG in the prevention of infection by these intracellular bacteria is not recommended. In countries where BCG is not part of the vaccination schedule, nontuberculous mycobacterial respiratory infections are more frequent, particularly in patients with cystic fibrosis and bronchiectasis of other etiologies.1 Protection against C. albicans and other bacteria such as S. pneumoniae and H. influenzae possibly occur through cross-protection.35
Epidemiological studies in several countries have shown that the BCG vaccine was associated with a reduction in childhood morbidity and mortality beyond what would be expected for protection against TB. There is certainly a bias involved, as vaccinated children are generally the healthiest, but in some studies, the reduction in mortality was so significant that it could not be explained by this bias.29
Protection against respiratory tract infections, but not against digestive or urinary tract infections, without adverse effects, was identified in subjects over 65 years of age who received BCG. Protective beneficial actions against viral infections such as H1N1, yellow fever, respiratory syncytial virus, human papillomavirus, metapneumovirus, and herpes simplex virus have been demonstrated in several publications.35 In some of these, it was demonstrated that the levels of antibodies against the studied virus did not differ between the group that received BCG and the group that received a placebo, indicating that the observed beneficial effect was due to trained immunity.1
BCG action in preventing SARS-CoV-2 infection, as well as in reducing morbidity and mortality, has been studied since the beginning of the COVID-19 pandemic. The results to date are conflicting and do not allow a conclusion to be drawn about its usefulness in fighting this infection.36 Many of these studies compare epidemiological data between countries in which BCG is routinely applied and countries that do not apply it, failing to consider the multiple factors that can interfere with the data, such as access to health services, socioeconomic status, age group, and population density, among others.37
The immunomodulatory action of BCG has been studied in some immunologically mediated, autoimmune, or allergic diseases. The application of ID BCG promoted a reduction in demyelination lesions and less development of new lesions in patients with multiple sclerosis documented by magnetic resonance imaging, according to studies carried out from the last decade of the 20th century onwards. In addition, there was evidence of a reduction in Th17 and an increase in Treg, with a reduction in T CD45+ cells in the cerebrospinal fluid (CSF) of these patients.18 There has been no success in preventing the progression of autoimmune diabetes mellitus (DMT1) in humans with the use of BCG in the neonatal period or given soon after the diagnosis. However, more recently, beneficial metabolic effects have been demonstrated, with the stabilization of glycated hemoglobin, as well as an increase in the number and function of Treg cells and an increase in autoreactive T cell apoptosis in patients with type 1 DM who received BCG.1,18 Conflicting results were obtained in studies that sought to associate a lower frequency of atopic diseases, such as asthma, rhinitis, and atopic dermatitis, or food allergy in individuals who received BCG. Two systematic reviews, however, showed a protective effect of BCG against asthma.1
Research on the use of BCG as a type of immunotherapy for malignant tumors originated from the observation of a lower incidence of bladder cancer in patients with TB in 1928. Many studies followed with different types of tumors, until the first publication that demonstrated the success of intravesical BCG therapy in 1976.1
Studies that seek to associate the use of BCG with a lower incidence of different types of cancer, solid and hematological, carried out since the 1970s, have disclosed conflicting results. The use of BCG by scarification or by intradermal (ID) route produced less significant responses than its intralesional application for the treatment of stage III melanoma, which resulted in a complete response limited to 19% of cases. In the case of prostate cancer, studies have shown better survival of patients treated with BCG ID or by intraprostatic route but showing a worse quality of life. Studies with the application of intrapleural or ID BCG for the treatment of small cell lung cancer have not found a positive impact on survival.1
One cannot fail to mention the risks that BCG, a live microorganism vaccine, poses to patients with inborn errors of immunity, particularly severe combined immunodeficiency, chronic granulomatous disease, and defects with Mendelian susceptibility to mycobacteria. There are also reports of autoimmune phenomena following BCG, as it can occur with TB, such as arthritis following intravesical BCG for bladder cancer therapy.12 The role of the enhanced innate immune response produced by trained immunity in the development or maintenance of autoimmune or inflammatory diseases remains controversial.38
ConclusionsThe discussion about heterologous protection promoted by BCG, most likely due to the stimulus of trained immunity, has the great benefit of pointing to the possible relevance of active immunizations in cross-protection against several infections.
The importance of BCG in the prevention of TB and in the treatment of bladder cancer without muscle invasion is now fully established.
Considering the centrality of the immune response in the control of infections by different types of microorganisms, as well as in the control of autoimmune manifestations and cancer cells, the capacity of BCG to stimulate the innate and adaptive immune responses epigenetically and metabolically is at the heart of the immunological rationale for its use in addition to the traditional indications. However, despite a great deal of evidence obtained through in vitro studies, and some indirect epidemiological and direct clinical evidence, more robust evidence of its in vivo efficacy is still needed to justify its use in clinical practice, beyond what is recommended by the NIP and in the treatment of bladder cancer.