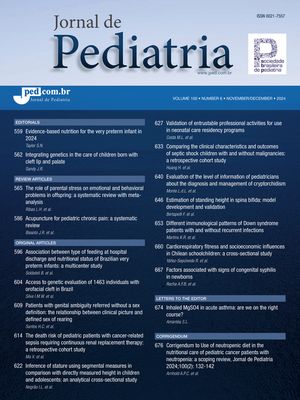
To review in the literature the environmental problems in early life that impact the respiratory health of adults.
SourcesNon-systematic review including articles in English. Search filters were not used in relation to the publication date, but the authors selected mainly publications from the last five years.
Summary of the findingsIn this review, the authors present the exposure pathways and how the damage occurs depending on the child's stage of development; the authors describe the main environmental pollutants - tobacco smoke, particulate matter, air pollution associated with traffic, adverse childhood experiences and socioeconomic status; the authors present studies that evaluated the repercussions on the respiratory system of adults resulting from exposure to adverse environmental factors in childhood, such as increased incidence of Chronic Obstructive Pulmonary Disease (COPD), asthma and allergies; and, a decline in lung function. The authors emphasize that evidence demonstrates that adult respiratory diseases almost always have their origins in early life. Finally, the authors emphasize that health professionals must know, diagnose, monitor, and prevent toxic exposure among children and women.
ConclusionThe authors conclude that it is necessary to recognize risk factors and intervene in the period of greatest vulnerability to the occurrence of harmful effects of environmental exposures, to prevent, delay the onset or modify the progression of lung disease throughout life and into adulthood.
Environmental pollution is recognized as a global health emergency affecting high- and low-income countries. Exposure to environmental risk factors contributes to the onset and progression of respiratory diseases.1
Data from the World Health Organization (WHO) show that 4.2 million deaths annually are attributed to exposure to environmental pollution.2
The environmental conditions in utero and in early childhood are critical for the individual to achieve potential growth and lung function and will determine a child's future. Children exposed to pollutants from the prenatal period are particularly vulnerable to certain risks.3 The exposure occurs through the interaction with the environment during the natural process of growth and development. Adverse environmental exposure in this critical period of life will determine structural and functional changes in the respiratory system. The association of environmental exposure, prenatal or perinatal, with adverse birth outcomes, such as preterm delivery, lower birth weight, and lung development defects, is well documented.4
Epidemiological studies demonstrate that respiratory diseases that manifest in adults most often have prenatal and early life origins.5-7
Adverse childhood exposures affect adult respiratory health resulting in an increased risk of non-communicable chronic respiratory diseases such as chronic obstructive pulmonary disease (COPD) and asthma; pulmonary aging with an accelerated or premature decline in lung function; and reduced life expectancy.3,8,9
Environmental pollution, indoor pollutants (especially tobacco exposure), traffic-related pollution can cause neonatal problems and respiratory diseases that will have repercussions in adulthood. There are many studies showing associations between exposure to environmental tobacco in the fetal period causing a higher risk of prematurity and early decline in lung function in adults.10-13
In this review, the authors will address how exposure to environmental hazards early in life can have an impact on adult respiratory health. The effects resulting from respiratory infections and late exposure and into adulthood will not be addressed.
EpidemiologyIn the pediatric age group, exposure tends to be daily, persistent, and in most cases cannot be avoided. In 2021, 91% of the world population will live in places with pollution levels above the limits recommended by the WHO guidelines. The intensity of exposure to air pollutants varies depending on geographic regions and household conditions. Low- and middle-income countries have higher exposures. Half of the world's population is exposed to indoor smoke from fires and wood stoves, responsible for up to 1.5 million premature deaths annually.14 In Africa, 80% of children live in homes with dirty energy sources. About 12.9 million women are potentially exposed to toxic electronic waste that puts them and their children at risk.2,15,16
In 2017, 42% of the world population was exposed to fine particulate matter (PM2.5) above that recommended by the WHO, and 43% were exposed to ozone. In Europe, in 2021, 84% of the population was exposed to PM2.5 and 9% to Nitrogen dioxide above the limits of this guideline.8
It is estimated that 24% of deaths are associated with environmental conditions.1 In Europe, there are 400,000 premature deaths/year caused by air pollution. It is estimated that the average life expectancy of a European is reduced by one year due to air pollution. A considerable proportion of premature deaths in European cities could be avoided annually by reducing air pollution concentrations. It must be considered that any death and loss of years of healthy life are not acceptable as both are preventable. Furthermore, there is no safe exposure limit below which air pollution is not harmful to human health.8
The child and the environmentPollutants come from air pollution; inadequate water, sanitation, and hygiene; hazardous chemicals and waste; radiation; as well as emerging threats such as electronic waste.16
Children are particularly vulnerable to environmental pollution as they receive a proportionally higher dose than an adult exposed to the same environment and often the adverse effect is greater due to their lower capacity to metabolize and excrete the toxic substance.4 Newborns and young children have a higher respiratory rate and proportionally inhale a higher dose of pollutants than older children and adults in the same environment. When exercising outdoors, children are more exposed to air pollutants as they have a relatively larger lung surface compared to body size.3,4
Pathways of exposure to environmental insultsTransplacentalThe placental barrier has a protective function for the fetus, but it is not complete. Toxic substances inhaled by the mother or absorbed by the maternal oral mucosa that pass directly into the maternal bloodstream reaching the fetal circulation.3,4
Breast milkIt is the ideal food for babies, but it can contain harmful substances. Water-soluble chemical compounds and lipids can be transferred into breast milk, such as organochlorine pesticides, metals, solvents, etc.3,4
Ingestion of contaminated water and food and non-nutritive ingestionIt is part of a child's normal development to take objects and hands to the mouth, which can favor the ingestion of household dust, soil, and chemical contaminants present in the objects.3,4
CutaneousInfants and young children have a proportionally larger body surface area than adults and older children, with a larger area of exposure per kilogram of weight, and have immaturity in the protective functions of the skin and subcutaneous tissue. Also, they may have more uncovered areas of the skin surface and are in greater contact with particulate matter from household dust, soil, chemicals such as pesticides, and cleaning products.3,4
InhaledAir pollutants can accumulate in the airways or pass through lung tissues, depending on the size and chemical nature of the pollutants. Ultrafine particles can pass into the bloodstream and reach distal organs.3,4
The respiratory systemPulmonary morphogenesis begins in the embryonic period of fetal development, 3-4 weeks post-conception, while growth and maturation continue until the age of 18-20 years. Lung development is subdivided into five different periods of morphogenesis based on morphological characteristics: Pseudoglandular stage (6-17 weeks gestation) - Bronchi, bronchioles, and acinar tubules; Canalicular (16-26 weeks gestation) - acinar structures; Saccular (26-36 weeks gestation) - saccules and alveoli and cell differentiation and; Alveolar after 36 weeks post-conception and continues during somatic growth.4,17
From the 36th week after conception, there is greater growth and expansion of pulmonary acini and development of the distal capillary network. In this phase, the lung grows mainly due to septation, proliferation, and thinning of the alveolar walls, as well as the elongation and luminal enlargement of the conducting airways.4 The respiratory system is not fully developed at birth, and lung growth occurs mainly in the postnatal period. Adverse environmental exposure in the pseudoglandular phase interferes with the structural development of the airways, and later exposures can cause bronchial thickening and hyperresponsiveness after birth.4
How does damage to the respiratory system occur?The respiratory system is vulnerable to environmental exposure, which occurs both prenatally and postnatally, especially in the first 18-24 months of life.4,18
The degree of impact caused by adverse exposure varies and depends on the period of exposure. Sly et al. call this period the “window of susceptibility”.3 It would be the period in which a certain environmental exposure can cause structural or functional abnormality, resulting in impaired respiratory health. Occurring outside this period, the pollutant would have much less of an effect.3
Individual vulnerability to a given exposure can be influenced by the child's stage of development (prenatal, infant, child); type and route of exposure; genetic and epigenetic factors; comorbidities; nutritional status; and sociodemographic conditions. Transgenerational factors and preconceptions also have deleterious effects on lung development. Lung health can be affected by several mechanisms such as interruption of lung growth potential, modulation of function, modification of gene expression, and alteration of the pathophysiological mechanisms of the airways.3 Furthermore, the respiratory system does not work in isolation. Factors that cause dysfunction of the immune system, the central nervous system, may also have consequences for respiratory problems and diseases.3,4
What is the impact of environmental problems on respiratory health?The main consequences of environmental damage on adult respiratory health are associated with loss of lung function and diseases such as COPD and asthma.
Impairments to pulmonary functionThe assessment of lung function by spirometry, commonly presented as Forced Expiratory Volume in First Second (FEV1) and Forced Vital Capacity (FVC), allows indirect measurement of lung growth. Lung volumes increase from childhood to young adult with maximum values reached at 20-25 years of age, remaining on a plateau for around five years and then gradually decreasing with aging. This process is called the trajectory of lung function. This decrease of lung function varies between individuals and is impacted by genetic and environmental factors that, in turn, influence both the development and progression of COPD.
Lung function early in life (at birth and at age 4-6) can predict the maximum lung volume a person will reach when they reach the plateau.19 In adults, low FEV1 is associated with greater morbidity and mortality.20 Low lung function early in life usually does not recover and is a risk factor for airflow obstruction into adulthood.21 In rare circumstances, there is potential for recovery. Harmful environmental exposures in early life may cause a reduction in the expected increase of lung function in early childhood and are associated with an increased risk of respiratory diseases in adulthood.
COPDCOPD is the main cause of chronic morbidity and is among the three leading causes of death worldwide. The Global Initiative for Chronic Obstructive Lung Disease (GOLD) defines COPD as persistent respiratory symptoms and airflow limitation due to airway and/or alveolar abnormalities, caused by exposure to harmful particles or gases and influenced by individual, genetic and environmental factors.22 Results from different trajectories or their combinations (assessed in young adults): abnormal at birth; failure to reach a normal spirometric plateau or accelerated decline in lung function.7,21,23 Exposure to tobacco is a risk factor for COPD, but there is evidence that non-smokers also develop the disease. Harmful events that occur in pregnancy or childhood have the potential to alter lung growth and increase the risk of developing the disease.6
AsthmaAsthma is a heterogeneous disease characterized by chronic airway inflammation. It manifests as wheezing, ventilatory difficulty and chest tightness, which vary over time and in intensity, associated with variable obstruction of the expiratory airflow. The obstruction can become permanent.24
Environmental exposures and adult respiratory healthThe main environmental pollutants, their characteristics, and the result of exposure on respiratory health are presented below.
Tobacco exposureTobacco exposure affects not just individual smokers, but those around them, including casual contacts in bars, and potentially especially but not exclusively in women, transgenerational adverse effects. Since tobacco contains many chemicals in addition to nicotine, it is only in the context of animal experiments that the authors can be certain which compound(s) is the causative agent. However, from animal data (below), it is clear that many adverse effects on the fetus are caused by nicotine. The role of e-cigarettes must also be considered in this context; they have some toxicities which overlap with tobacco, some effects which are unique to these devices, and many deliver high concentrations of nicotine. Thus the assumption must be that e-cigarettes are as harmful if not more so than conventional tobacco, unless and until proven otherwise – and it is for the vaping industry to prove they are safe, not for pediatricians to prove they are unsafe, just as Pharma have to prove the safety of a novel inhaled medication.25
Transgenerational effectsThe earliest exposure is from grand maternal smoking, presumably by an epigenetic effect on the germline, although this is controversial. Her own offspring will be more likely to smoke and more likely to have asthma in consequence. However, if she has a daughter, even if that daughter does not smoke, her children are more likely to have asthma. If she does smoke, and they also smoke, the effects on the children's lung function are much greater.26,27 Whether the same effect is seen in great-grand-children is unknown.
Antenatal effectsKnown effects of nicotine from animal workThe experimental administration of nicotine to different pregnant animal models has established the following:
- •
There is increased collagen type 1 and 3 mRNA and immunostaining in the developing airway and lung parenchyma; there was increased elastin mRNA but not protein.28
- •
MUC5AC is over-expressed; this is of particular interest in terms of later mucus hypersecretion, a chronic obstructive pulmonary disease trait that is increasingly recognized as important.29
- •
There is dysanaptic airway growth, the airways are abnormally long, and there is airway hyper-responsiveness (AHR) in the newborn period, independent of infection or allergen exposure.30 This is particularly important in that AHR in the newborn period is strongly predictive of later asthma and impaired lung function in human studies, and dysanaptic airway growth is associated with worse asthma outcomes in childhood and adult life.31
- •
There is a failure of secondary septation leading to early-onset emphysema.32 As yet unpublished studies measuring alveolar size using hyperpolarized Helium suggest that teenage offspring of mothers who smoked in pregnancy have abnormally large alveoli.
- •
There is the increased airway wall thickness and loss of alveolar attachments to the airway. These attachments stabilize airways by interdependence. This finding has been confirmed in early infant deaths of offspring of mothers who smoked in pregnancy.33
- •
(The exact components of cigarettes causing these abnormalities is not known)
- •
There is increased cord blood mononuclear cell reactivity to allergens in the offspring of smoking mothers.34
- •
There is reduced cytokine and Toll-like receptor function associated with antenatal tobacco exposure.35 Children who subsequently develop wheeze in the first year of life with either rhinovirus, respiratory syncytial virus, or other viral infection have reduced cord gamma interferon responses.36
In summary, the readout of tobacco exposure antenatally is:10
- •
Low birth weight, itself an independent risk factor for impaired lung function and subsequent asthma.
- •
Prematurity and small for gestational age birth. The effects of these two factors could be the subject of a whole review, and not all their effects are the same, but these patients have persistent airflow obstruction without evidence of Type 2 inflammation (unless there is coincidentally an atopic background). Interestingly, and relevant to data discussed below, there is more than associated respiratory morbidity associated with prematurity; early ischemic heart disease and cardiac failure are associations of bronchopulmonary dysplasia.
- •
Airflow obstruction at birth, also predictive of the later development of asthma.
- •
Altered immunological function at birth (and discussed in more detail below).
- •
Sensitization to the effects of later adverse stimuli such as occupational exposures.
- •
Increased likelihood of impaired lung growth and later accelerated decline in lung function.
- •
Premature cardiovascular and all-cause morbidity and mortality.
These implications may extend beyond mothers who smoke to non-smoking mothers who are passively exposed to tobacco. For example, Belgium's data showed very clearly that, as passive smoke exposure was reduced by a series of new laws, the rate of preterm delivery fell.11 This is a really important message; these and other data emphasize that smoking does not merely affect the smoker but also the innocent bystander. Pediatricians must be advocates to prevent these exposures.
Effects of smoke exposure in childhoodThe effects of smoke exposure on childhood respiratory morbidity are very well known and have been summarized elsewhere; pediatricians are fully familiar with these and know that smoke exposure must be minimized.35 Indeed, smoke-free legislation dramatically reduces asthma attacks in children. Recently, it has been shown conclusively that three environmental factors are associated with impaired lung growth and progression of preschool wheeze to school-age asthma; these are aeroallergen sensitization, attacks of severe wheeze, and exposure to tobacco smoke, of which only the last is readily modifiable.37 What is far less appreciated is the long-term consequences, both respiratory and systemic, of childhood smoke exposure, discussed in more detail below.
Lung growth and developmentA major recent advance in the field has been the use of mathematical tools to delineate the different growth trajectories objectively, letting the data speak for itself rather than imposing investigator bias. These have typically identified 4-6 trajectories, although it should be noted that at least some individuals appear to change trajectory in childhood. Thus combining the Manchester and Avon longitudinal studies, nearly 2,500 children, the four classes were persistently high, normal, below average, and persistently low, of which the persistently high group were least likely to have been exposed to tobacco.37 The Tasmanian cohort, extending from the first to the sixth decade of life, delineated six: persistently high, average, below average, persistently low, early below average with accelerated decline, and early low with accelerated growth and normal decline.38 The likelihood of chronic obstructive pulmonary disease (COPD, 46%) was highest in the below-average, fast decline group, and childhood tobacco exposure was one factor in this trajectory.
How adult respiratory morbidity is determined by early life events- •
Occupational asthma: although this is considered to be a pure adult disease, in fact this is not the case. An important study hypothesized that early life disadvantage sensitizes to occupational lung disease. The investigators used the RHINE III questionnaire on occupational cleaning in 13,499 subjects and found that early life disadvantage (which included maternal smoking) was associated with an increased risk of wheeze, ‘adult onset’ asthma (discussed below), and self-reported COPD in 2138 cleaners. The strongest effect was early life disadvantage.13
- •
“Late-onset” asthma: this is a condition much talked about by adult physicians, but it actually does not exist. In the Tucson study, the prevalence of Physician diagnosed asthma and airway function was determined at age 22 years; and 25% of active asthma were newly diagnosed at that age (71% women).39 However, when the prospectively obtained data were examined, AHR and low airway function at age 6, wheeze onset age 3-6 years, and persistent wheeze through first six years of life all predicted “newly diagnosed” asthma at age 22; and most of these factors are unequivocally associated with early tobacco exposure. It is very clear that this is not ‘late-onset asthma” but “late recrudescent airway disease”, and the roots need to be sought in early life.
The two routes to COPD are accelerated decline in lung function having reached a normal plateau age 20-25 years (7% risk of COPD), and normal rate of decline from a reduced plateau (26% risk).40 The latter is clearly the result of early life factors, of which tobacco exposure is an important factor. In the classic Fletcher and Peto paper, accelerated decline was attributed to current smoking, which could be halted if the patient abstained from tobacco.7 Although, of course, smoking is a “BAD THING”, in many but not all COPD cohort studies, there is no effect of current smoking on decline in spirometry. In an illuminating study in the SPALDIA and ECRHS cohorts, early disadvantage (including maternal smoking) was associated with a more rapid decline in spirometry; as with the Tucson study, personal smoking effects were potentiated by maternal smoking.5,41 Similar findings were reported by the ECFS study, wherein accelerated decline in lung function was associated with (amongst other exposures) early tobacco exposure. Importantly, the idea that was just stopping adults smoking, beneficial though that would be, will prevent COPD is simply not true. In many cohorts, there is no signal for current smoking, causing an accelerated decline in spirometry.42
More than just lungs: is reduced lung function the “canary in the mine”?Early low lung function, for which tobacco exposure is probably the main cause, is unsurprisingly associated with bad long-term respiratory outcomes (above). But it is also very clear that a reduction in the first second forced expired volume (FEV1) means more than just a bad respiratory outlook. It is associated with early and increased cardiovascular and all cause morbidity and mortality, and this is unsurprising when the multi-organ adverse effects of nicotine are considered.12 Also, the same study reported a transgenerational effect on spirometry – low parental FEV1 was strongly predictive of offspring FEV1 (r2 = 0.28), another example of how the smokers poison not merely themselves, but those around them and future unborn generations.12
Air pollutionAir pollution is the presence of one or more contaminants in the atmosphere, such as dust, gas, mist, odor, smoke, or vapor, in an amount and duration that can be harmful to human health. Pollutants produce inflammation, oxidative stress, immunosuppression, and mutagenicity in cells, which can affect other organs, causing diseases.15 The pollutants present in the atmospheric air originate mainly from human activity in urban areas, originating from automotive vehicles, and, in non-urban areas, biomass burning. Sources of natural environmental exposures are derived from accidental fires, sandstorms, and electrical lightning that can generate nitrogen oxides (NOx).15 Pollutants have different physical and chemical characteristics depending on the type of pollution source. The so-called primary ones are those emitted directly into the atmosphere and produced mainly by industries, thermoelectric plants, and automotive vehicles. The most frequent are sulfur dioxide (SO2), nitrogen oxides (NOx, NO, and NO2), metals, carbon monoxide (CO), volatile organic compounds (VOC), and particulate matter (PM). Secondary calls are those originating from chemical reactions between vehicular and industrial emission pollutants, such as NOx, which react with volatile organic compounds (VOCs) emitted by vehicles, solvents, and industry. The process takes place in the presence of ultraviolet rays from sunlight, thus giving rise to ozone.15 Nitrogen dioxide (NO2), a toxic gas that causes significant inflammation of the airways, originates from combustion processes (heating, power generation, and engines in vehicles and ships). Sulfur dioxide (SO2) is a gas produced by burning fossil fuels (coal and oil) for domestic heating, power generation, and vehicles.
Prenatal and early life exposure to pollutants, including nicotine and air pollution, leads to impaired lung function, which remains largely altered until the sixth decade of life. Maternal exposure to air pollutants is associated with decreased lung function during childhood that may be further reduced in adults and consequently lead to COPD.38 Both outdoor and indoor pollution increase the chance of COPD.
Fetuses born to mothers exposed to environmental pollutants are at increased risk for adverse birth outcomes such as intrauterine growth restriction, prematurity, low birth weight, and fetal lung development defects.43,44 These adverse outcomes are associated with the development of late-onset respiratory diseases and reduced lung function, in addition to increasing the susceptibility to the harmful effects of other pollutants. Maternal exposure to Benzene and NO2 in the third trimester of pregnancy determine decreased lung function at school age.45
Babies who grow up breathing polluted air, even at levels below EU regulations, have lower lung function from infancy through adolescence, and the results are worse in children with asthma. Part of the risk of exposure to pollution can be reduced if babies are breastfed for at least 12 weeks.46
The reduction in the increase in lung function is also linked to NO2 exposure.47 Children exposed to higher levels of NO2 and PM2.5 are five times more likely to have reduced lung function than children living in areas with lower levels.48 Another study demonstrated that decreasing environmental NO2 and PM2.5 significantly decreases the risk of reduced lung growth.49
Particulate matter (PM)Mixture of droplets or particles suspended in the air, composed of hundreds of organic or chemical substances such as ozone, nitrogen oxides, SO2, dust, soot or smoke, from coal and natural gas plants, cars, fires, unpaved roads and construction sites. Particles with an aerodynamic diameter less than 10 µm (MP10), such as sand, dust, and pollen, usually deposit in the nasopharynx and are less toxic. Fine particles, smaller than 2.5 µm (PM2.5), originating from combustion and ultra-fine particles (less than 0.1 mm), emitted by diesel engines, can penetrate tissues and organs, causing greater risk to health. Prenatal exposure to PM10 is associated with reduced lung function.50 The effects may be even greater in children born prematurely.51
External pollutantsOriginating from emissions from agricultural industries and activities, vehicles and air traffic, products used in agriculture, war, accidents, and fires, or from natural events such as volcanic eruption, forest fires, manufacturing, and distribution of chemical materials. Individuals exposed in the first year of life or during pregnancy to the Great Smog of London in 1952 were more likely to have asthma (9.53%) in adulthood.52 Another study showed that a higher incidence of asthma at age 20 years was associated with greater exposure to all pollutants at birth, including PM10 and NO2.53
Household pollutantsThe main source is cigarette smoke (above). Other sources are wood-burning, charcoal, crop residues, animal manure, and kerosene used as a fuel source for cooking and heating. Burning these materials combined with inefficient stoves leads to the emission of high concentrations of gaseous and particulate pollutants, methane, carbon monoxide, polyaromatic hydrocarbons (PAH), and VOCs. Other factors include products eliminated by air conditioning, cleaning products or flavorings from environments with oils, use of sprays and aerosols, and windows and doors open, allowing ingress of external pollution and the effects of pollution. Household pollutants are more problematic for infants and young children as they stay longer within the home. The quality of indoor air, including exposure to fungi, is associated with the onset of asthma. Individuals who were exposed to indoor pollutants during the first three years of life with early specific sensitization (mites, cat or dog) are at increased risk of developing asthma at 20 years of age. The effect is greatest if associated with pre-and postnatal exposure to tobacco smoke.54 Individuals with early mite sensitization progress with worsening lung function that is lower at school age than at age five years and remains low until age 26.55 Data from developing countries show that in individuals with COPD, 35% were early exposed to cigarette smoke and indoor biomass combustion. Childhood exposure to indoor coal pollution increases all-cause mortality, with a greater impact on respiratory causes, and the risk is greatest when associated with exposure to fuel.56
Air pollution associated with traffic (TRAP)Automobile exhaust releases pollutants into the environment. There is an increased risk of asthma in children exposed to external pollutants such as ozone, sulfur dioxide, particulates, and nitrogen oxide. The highest incidence of asthma also occurred in adults who had been exposed to traffic pollution during the fetal period. Children living near major highways in contact with external pollutants (PM, O3 and SO2 from vehicular traffic) have a lower FEV1 than those living further away.57 This reduction in FEV1 is associated with the development of COPD. Children who spent more time outdoors in areas with high levels of ozone had reduced lung function, while those who grew up in environments with low levels of pollution had greater lung function. Traffic-based pollution is associated with many childhood morbidities and an increased risk of adult COPD and lung cancer. A meta-analysis showed that childhood exposure to TRAP (Black Carbon, NO2, PM2.5, PM10) contributes to the development of asthma.58 Another study demonstrated that improvements in air quality were associated with statistically and clinically significant positive effects on lung function growth in children.49
Asthma and allergiesThe immune system is not yet fully developed, so there is an increased risk of respiratory infections and asthma. In asthmatic patients, exposure to air pollutants can cause oxidative damage to the airway, leading to inflammation, remodeling, and an increased risk of sensitization. In adults, the accumulated effect of exposure to environmental pollutants can contribute to new-onset asthma.59
Household and outdoor pollutants, including O3, CO, NO2, SO2, PM10, PM2.5, dust mites, pollen, pet dander, and smoke, contribute to more severe allergic responses. One study showed that 11% of asthmatic children with abnormal lung function had pulmonary function with fixed obstruction in adulthood compatible with COPD.60 Exposure to traffic pollution contributes to 13% of the global incidence of asthma in children.61
Patients with COPD have more asthma-like symptoms in the early years of life, as demonstrated in three cohorts over several decades.37 Having severe childhood asthma was the strongest predictor of COPD at age 50.62 In a study of 3290 mother-infant pairs, 23% had asthma-like symptoms in childhood, and these individuals had more admissions and use of COPD medication in adulthood compared to those without asthma-like symptoms.63 Another study in adults aged 50 years showed that those with symptoms of severe asthma at 6-7 years were 32 times more likely to have COPD than those without symptoms. In this group, only 40% were smokers suggesting that other harmful exposures may have contributed to the disease.64
Persistently low or rapidly declining lung function and development of COPD are associated with risk factors present in childhood (maternal and paternal asthma, maternal smoking, childhood asthma, and respiratory infections).38,65 In addition, preterm (< 35 s) and underweight (< 2,000 x < 2,100 g) girls are at higher risk of COPD and asthma than preterm boys.66
Belgrave et al. studied the trajectory of lung function from childhood to adulthood in 2436 individuals and identified four trajectories: persistently high, normal, below average, and persistently low. Children with a persistently low trajectory were more likely to recurrent wheezing, including severe exacerbations of wheezing, early allergic sensitization, and exposure to tobacco smoke.37 Avoiding these exposures could decrease the chance of lower lung function in adulthood.37
A study of 69,365 individuals identified that exposure to prenatal and early life smoking is a risk factor for COPD. Low birth weight, lower respiratory tract infections, recurrent wheezing, and childhood asthma also appear to be important determinants of the development of COPD.67 Parental asthma and smoking, preterm birth may be risk factors for the development of COPD.
Exposure to smoking and e-cigarette during pregnancy possibly impair the maturation of the immune system.68,69 Severe respiratory infections in childhood are associated with decreased lung function and increased respiratory symptoms in adults.60
Adverse childhood experiencesAdverse Childhood Experiences (ACEs) have a prevalence of around 10% and are currently a global public health and social welfare concern. EAIs include harm that affects children directly (neglect, physical, sexual, or emotional abuse) and indirectly through their living environments (conflict with parents, substance abuse or mental illness) and are powerful predictors of harmful behavior to health in adults. A systematic review and meta-analysis of studies measuring the associations between various (ACEs) and health in over 18s found that individuals who had at least four adverse experiences during childhood or adolescence were twice as likely to be smokers and to have respiratory diseases than those who did not have ACEs.70 Another meta-analysis has shown an association between ACEs and the occurrence of cancer in adults, including lung cancer.71 One hypothesis about the mechanism linking ACEs and cancer development in adults would be the possible association with risk behaviors such as smoking, physical inactivity, poor diet, and use of psychoactive substances.71
Socioeconomic factorsThere is a complex interrelationship between socioeconomic, ethnic/racial factors and health outcomes. Poverty and nutritional disorders (malnutrition and obesity) can increase the adverse effects of exposure and the risk of diseases attributed to a pollutant. Socioeconomic factors can influence the ’child's birth weight (which impacts lung growth and development) which increases susceptibility to the disease.4 Lange et al. demonstrated that 50% of individuals with COPD had abnormal lung growth and development (failure to reach the normal plateau, above), with the functional decline being within normal limits.40 Another study demonstrated synergism between overcrowding at home, smoking, and respiratory infection resulting in worse lung function at 43 years of age.49 However, it is not well defined to what extent the socioeconomic situation may reflect exposure to indoor and environmental pollutants, overcrowding, or other factors related to social conditions.
“Childhood disadvantage factors”They appear to be as important or more important than heavy smoking in predicting lung function in adulthood. Svanes et al. showed that children with a score indicating greater handicap had a more accelerated decline in lung function.42 The effect on more vulnerable individuals, such as preterm infants, may have even greater repercussions.42 Reduced lung function at birth and less-than-expected increased lung function in early childhood are associated with an increased risk of respiratory disease. Children of mothers exposed to CO have lower lung function at 30 days of life, and the effect is greater in girls.72 Dratva et al. studied 12862 adults aged 28 to 73 years and demonstrated that early exposure to certain factors (born during the winter season, mothers >31 years at delivery, with ≥ 2 younger siblings or exposed to maternal smoking) can predict a further decline in lung function and that the effect on decline in FEV1 was as significant as the effect of smoking.5 The greatest decline in FEV1 was in winter-born individuals with older mothers and mothers who smoked or had younger siblings. These same authors noted that if the mothers were older or smokers, there was a potentiation of the effects of smoking on the decline in lung function.5
In summary, in this review, the authors present evidence that early lung exposure to environmental pollutants (tobacco smoke, particulate matter, air pollution associated with traffic) and adverse childhood experiences and socioeconomic status cause an increased incidence of Chronic Obstructive Pulmonary Disease (COPD), asthma and allergies; and decline in lung function. There is evidence demonstrating that adult respiratory diseases can almost always have their origins in early life. It is emphasized that health professionals must know, diagnose, monitor, and prevent toxic exposure among children and women. By recognizing these risk factors, early interventions should be implemented to reduce the progression of lung disease throughout life and into adulthood.
How to prevent respiratory problems resulting from environmental exposure?In Table 1,5,16,24,70,73,74 interventions that the pediatrician can perform are listed below.
Pediatric intervention.
|
- •
Reducing the emission of pollutants, including air pollution and tobacco, is the best way to avoid damage to the respiratory system. In Europe, reducing air pollution by PM2.5 and NO2 could prevent 6 and 4% premature deaths annually, respectively.8
- •
Create alternatives for transport, industries, and energy sources with a reduction in the emission of smoke and toxic gases, and particulate matter.
- •
Afforest urban areas with minimally allergenic trees.
- •
Properly dispose of electronic waste.
- •
Implement local and international measures such as the WHO Global Strategy on Health, Environment and Climate Change.
Most children are exposed to some pollutants, and the home is the place where exposure is more intense and frequent, especially exposure to tobacco smoke. Exposure to environmental hazards early in life increases the risk of acute and chronic respiratory diseases. Future longitudinal studies are needed to assess the effect of specific exposures early in life, individual and collective prevention measures, behavioral and pharmacological interventions, and the impact on adult respiratory health. Pediatricians and the whole society must work together to identify risk factors, reduce avoidable exposures, protect the developing respiratory tract to reduce lifelong worsening or onset of disease and lung problems.